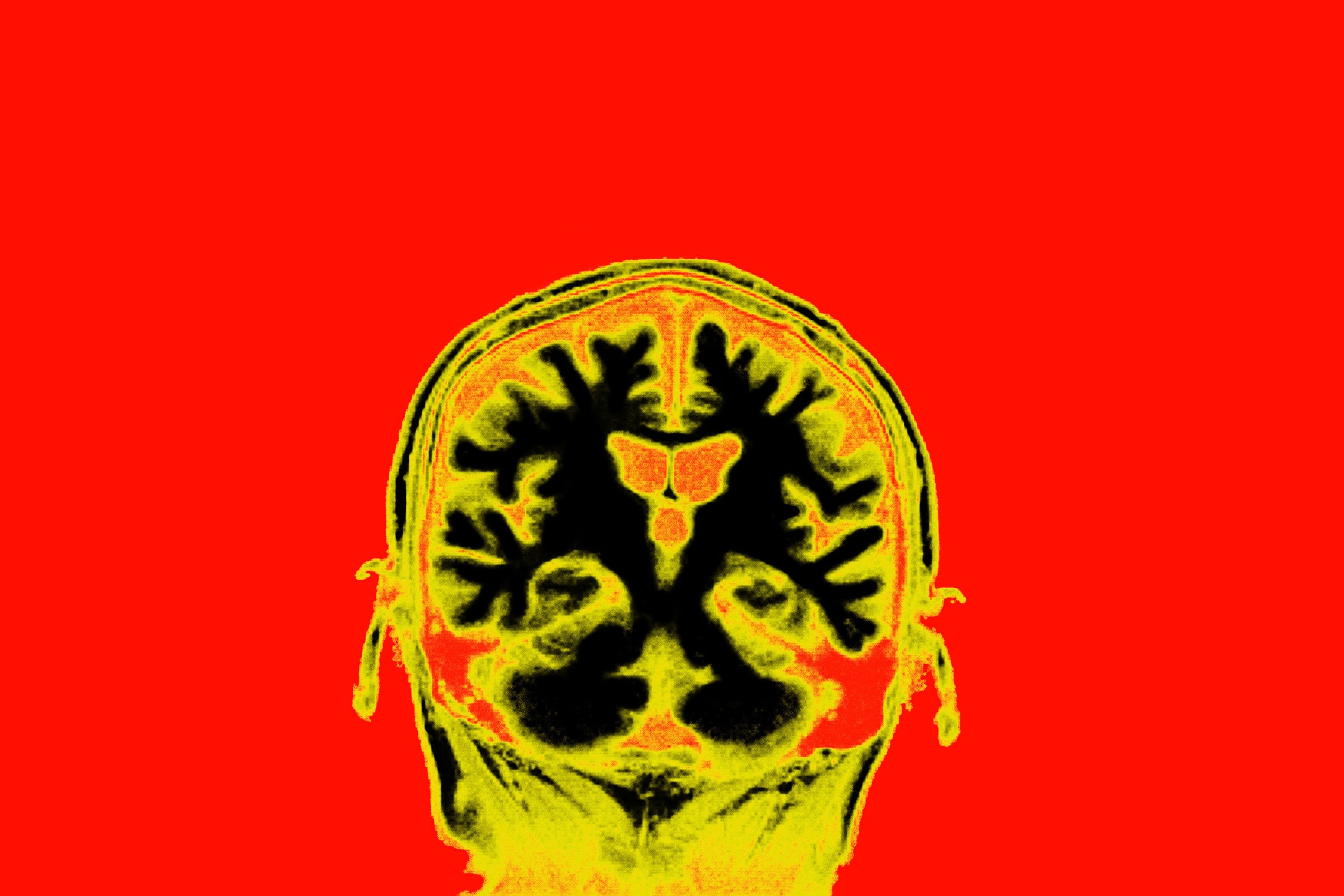
Every thought that crosses your mind has, literally, crossed your mind, as millions of neurons in different parts of the brain chatter with one another. Photograph: Getty Images
For the first time, researchers, including the co-director of HST, were able to observe, in extra-fine detail, how neurons behave as consciousness shuts down.
Max G. Levy | Wired
When you are awake, your neurons talk to each other by tuning into the same electrical impulse frequencies. One set might be operating in unison at 10 hertz, while another might synchronize at 30 hertz. When you are under anesthesia, this complicated hubbub collapses into a more uniform hum. The neurons are still firing, but the signal loses its complexity.
A better understanding of how this works could make surgery safer, but many anesthesiologists don’t use an EEG to monitor their patients. That bugs Emery Brown, who does monitor his patients’ brain patterns when they are under. “Most anesthesiologists don't think about it from a neuroscience standpoint,” says Brown, who is a professor of computational neuroscience at MIT and of anesthesia at Harvard Medical School, as well as a practicing anesthesiologist. Brown is also the co-director of HST, and also the associate director, MIT Institute for Medical Engineering and Sciences (IMES). IMES is HST's home at MIT. For the past decade, he has studied what happens to brains when their owners are unconscious. He wants to know more about how anesthetics work, and to track fine-grain signatures of how neurons behave when patients are under. He wants to be able to say: “Here's what's happening. It's not a black box.”
“And once you understand how to read these patterns, and you understand the neurophysiology behind them, you can dose your drugs better,” Brown says. “You're using physiology to take better care of your patients.”
In a study published in April in the online journal eLife, Brown’s team used electrodes to study the neurons deep inside the brains of monkeys undergoing anesthesia. The work shows, for the first time, how individual neurons in multiple regions of the brain respond as they become flooded with the sedative, and that their impulses slow by 90 to 95 percent. By eavesdropping on the brain’s chatter in different states, the team got an inside look at how consciousness emerges and recedes—and how doctors could better control it.
Every thought that crosses your mind has, literally, crossed your mind, as millions of neurons in different parts of the brain chatter with one another. “Your brain is a very rhythmic machine,” says Earl K. Miller, a professor of neuroscience at MIT’s Picower Institute who co-led the work with Brown. “It does this at all frequencies, from 1 hertz to up to 100 hertz or more.” Brain waves recorded from the scalp on an electroencephalogram, or EEG, show the cross talk of neurons collectively firing waves of electrical impulses across the outermost regions of the brain, or the cerebral cortex, which is typically seen as the control center.
Consciousness emerges from that dialog. “Sights, sounds, feelings, are all operating together to create this unified experience of what we're doing, how we're feeling, what we're thinking in a given moment,” says Miller. This, essentially, translates into an awareness of one’s own mind and the surrounding world—consciousness. The precise process of how neural activity translates to individual perception and thought is still not understood, but one way to explore what those neural circuits are doing to produce consciousness is to observe what’s happening to neurons when it switches off.
“One of the most interesting questions is how we experience cognition—how we have conscious experiences,” says Laura Colgin, a neuroscientist and director of UT Austin’s Center for Learning and Memory, who was not involved in the study. “Looking into general anesthesia as a window to understanding conscious experience is a really cool approach.”
Anesthesia basically tells your neurons to shut up. Propofol, the common anesthetic used in this study, sticks to proteins called GABAA receptors, making it harder for the cells to fire electrical impulses.
In earlier studies on brain implants in rodents and EEG readings from humans, Brown showed that propofol disrupts communication in the cortex. But to push the science further, he and Miller wanted to record different regions simultaneously as an animal slips in and out of consciousness. They wanted to use implanted electrodes to listen to individual neurons changing their tunes to get at how—and where—the brain’s complex communication breaks down under anesthesia. For their new study, they implanted 64-channel microelectrodes into four rhesus macaque monkeys. These were stuck into four sections of their brains: three regions of the cortex and the thalamus. Those three cortical regions are the frontal, temporal, and parietal lobes, which are associated with thinking, auditory processing, and sensory information, respectively. The thalamus is about the size and shape of a quail egg and sits deep in the brain, relaying info all around the cortex.
The scientists hit Record on the electrodes before flowing the first bit of propofol, and then they watched as the monkeys slipped into unconsciousness. “The drug goes everywhere, and it gets there in seconds,” Brown says. Brain waves slowed to a crawl. (Neurons in a healthy, awake brain spike about 10 times per second. Under propofol, that frequency falls to once per second or less.) Brown wasn’t surprised; he’d seen these types of slow oscillations before in other animals, including humans. But the deep electrodes could now answer something more precise: What exactly was going on among the neurons?
Normally, neurons chitchat by pulsing together. “Kind of like an FM radio,” Miller says. “They’re on the same channel, they can speak to one another.” Millions of neurons communicate this way, at many different frequencies. But now, the usual wealth of frequencies morphed into one low rhythm—a strange bit of harmony. Higher frequencies went away, and neurons were left communing on a low-frequency channel. It’s as if the sounds of a lunchroom packed with kids speaking in loud groups, quiet one-on-ones, and everything in between, just collapsed into one deep hum.
According to Brown, the less frequent spikes of neural activity during anesthesia are actually more coordinated than in any other mental state. Whether you’re alert, reading, sleeping, or meditating, your brain waves are chaotic and tough to parse. But no signal is as clear and rhythmic on an EEG as anesthesia. And, critically, he believes, it’s this uniformity that undermines consciousness. That lunchroom chatter from an alert brain seems like noisy chaos, but it’s actually a coherent language of memories, feelings, and sensations. The hum of anesthesia is clear, but it’s an information desert.
“Propofol comes along like a sledgehammer,” Miller says, "and just knocks the brain into this low-frequency mode where none of that is possible anymore."
Miller and Brown suspected that the thalamus would be especially important for reinstating the rich chaos of being awake. One existing theory suggests that, in order to produce consciousness, this small nub syncs up the various rhythms of the cortex. If the thalamus stops working, the theory goes, cortical waves can’t match their rhythms to communicate cohesive thoughts. “And communication is everything in consciousness,” Miller says.
Once they had observed that anesthesia flattened communication from the thalamus, the researchers wanted to see if stimulating that brain area would bring back signs of conscious activity. Previous work has shown that deep brain stimulation can restore some limb control to a person with a traumatic brain injury, as well as the ability to eat. Still, the idea is new. “It was a bit of a gamble, a long shot,” Miller says.
In a second line of experiments, the researchers stimulated the thalamus with electrodes, using current comparable to what people receive as a deep brain stimulation treatment for Parkinson’s disease. (This is painless, since the brain doesn’t have any sensation at all, even without anesthesia.) The monkeys blinked. Their heart rates went up, and their limbs moved. Neuron firing rates in some parts of the brain jumped back up to over three spikes per second. Low rhythms switched to a richer set of frequencies, indicating more normal chatter. In other words, the animals and their neurons behaved more like they do during consciousness, even though they were still bathing in a powerful anesthetic. That activity faded a few minutes after the current switched off. “We were able to partially restore consciousness and a conscious-like cortex,” Miller concludes.
Last year, Michelle Redinbaugh, a graduate student who researches consciousness at the University of Wisconsin-Madison, reported anesthetized macaques moving their bodies and faces, and having higher neuron spike rates after receiving similar stimulation in their thalamus. She thinks the new experiment indicates that the thalamus plays a profound role in our ability to form complex thoughts, and she believes it deserves more study. “This is further evidence that this is real, this is powerful, and it's something that more people need to look into,” she says.
Miller and Brown's work could make anesthesia safer, by allowing anesthesiologists who use the EEG to more precisely control drug dosages for people who are unconscious. And for some experts, a hope for the distant future would be to use the electrical stimulation of the thalamus to jump-start consciousness during operations, or after serious brain injuries or comas. Meanwhile, Miller hopes these results will help unlock one of the big mysteries of neuroscience: how skull-bound jelly blobs and complex neural interactions create our experience of the world.
* Originally published in Wired magazine.